Nanomechanics Lab
Nanomechanical cantilevers and membranes are exquisite sensors for minute forces, below one attonewton (1 aN = 10-18 N) at cryogenic temperatures. Our group is exploring nanomechanical force sensors for detecting and imaging nuclear spins with nanometer resolution. An ultimate goal of such a “nano-MRI” technique is the realization of a microscope that can directly image the three-dimensional structure of individual biomolecules
Magnetic resonance force microscopy (MRFM)
A magnetic resonance force microscope (MRFM) is a very sensitive variety of the scanning force microscope, capable of detecting the weak magnetic forces from nuclear or electronic spins. MRFM was conceived in the 1990’ies with the vision of pushing the spatial resolution of MRI (limited to micrometers-millimeters) down to the nanometer and possibly the atomic scale. At the heart of the MRFM instrument are a nanomechanical force sensor, a strong magnetic field gradient, and radio-frequency pulses to induce coherent spin inversions.
Magnetic resonance force microscopes are home-built instruments. The combination of cryogenic temperatures, high vacuum, magnetic fields of several Tesla, radio-frequency pulses, laser interferometry or cavity optomechanics, nanoscale scanning motors, and ultrasensitive mechanical elements results in a highly complex experiment.
By scanning a sample over the nanomagnetic tip, a three-dimensional image of the sample’s spin density can be recorded. This image represents an MRI scan of the sample, but with a much higher resolution compared to clinical instruments. In 2009, MRFM was used to generate three-dimensional MRI images of single virus particles at 5-10 nm spatial resolution. In our group, we attempt to demonstrate “Nano-MRI” on selected biological model systems. Efforts include speed-up of image acquisition and reconstruction, and new types of image contrast.
2009 Virus Imaging Paper: DownloadPNAS 106, 1313 (2009) (PDF, 2.2 MB)vertical_align_bottom
Useful Review: DownloadNanotechnology 21, 342001 (2010) (PDF, 893 KB)vertical_align_bottom
Nanomechanical membranes and strings
For more than a decade, the workhorse sensors for MRFM have been silicon cantilevers with a resonance frequency of 3-5 kHz. They reach a force sensitivity of a few aN per root Hz when cooled to liquid He temperatures. Recently, several groups started investigating the use of silicon nitride membranes as improved force sensors. These devices are typically in the MHz range and feature quality factors beyond 100 millions, which may allow the detection of forces below 100 zN. In addition, these membranes can be combined with laser cavities to reduce the readout noise down to the Heisenberg limit. Our lab is exploiting the exciting properties of silicon nitride membranes to realize a new generation of extremly sensitive scanning force microscopes, and to establish an alternative platform for nano-MRI.
Parametric resonators
Driven nonlinear resonators can have multiple stable states that are potentially useful for logic operations. One famous example of such a device is the degenerate parametric oscillator, or ‘parametron’. It features two symmetric phase states that form a quasi-potential landscape with two attractors. Invented in the 1950’ies for the first electronic computers, the parametron experienced a revival over the last 10 years because it can be used to simulate the behavior of a single spin in the so-called Ising model. Coupled parametrons may therefore be a useful resource for quantum annealing simulations, neuromorphic networks, and time crystals.
In our group, we investigate the behavior of coupled parametrons in the presence of fluctuations. The coupling gives rise to complex stability diagrams with unexpected bifurcation topologies, which in turn influence the stochastic switching rates between different attractors. So far, we have been using systems based on (classical) mechanical and electrical resonators. Despite their simplicity, these experiments have revealed many surprising details. In the future, we plan to build small parametron networks from quantum-coherent optomechanical resonators. This work is done in close collaboration with Prof. O. Zilberberg and Dr. R. Chitra from the Institute for Theoretical Physics at ETH Zurich.
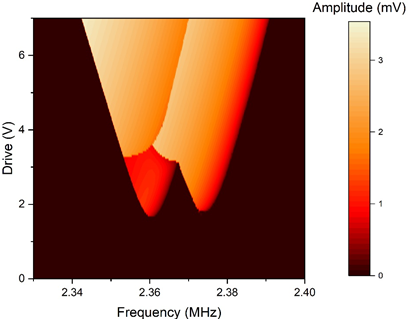